Planetary Defense
Right after the solar system had formed our planets performed some sort of housekeeping. Most of the primordial chunks of ice and rock that were not used up in planet-formation were ejected from the inner regions of the solar system. Some of those remnants (aka asteroids) orbiting the sun at specific distances were not so easy to get rid of, however. The so-called "main-belt" between Mars and Jupiter is such a region that still contains a vast number of asteroids as well as some comets. Interestingly, particular areas within the main belt were and are still cleansed rather quickly - the Kirkwood gaps, for instance. Those are caused by orbital resonances with Jupiter and Saturn. If main belt asteroids wander into such regions, it is possible that they are scattered toward the inner solar system. On their journey through the solar system asteroids can spend hundreds of thousands to millions of years in near Earth space. A tiny fraction of those asteroids may at some point collide with our planet. Asteroid impacts can have desastrous consequences, such as fireballs, earthquakes, and Tsunamis. Fortunately, astronomers are tracking more than 9 in 10 asteroids that can cause disasters on a global scale. Yet, we do still discover asteroids that could eradicate cities. Should an asteroid be discovered to be on an intercept course with the Earth we better do something about it! There are a number of ideas how to prevent potentially hazardous asteroids from impacting the Earth. One possible method of deflecting an asteroid's trajectory is a so-called "kinetic impact". A spacecraft would hit the asteroid at very high velocities, often tens of kilometers per second.
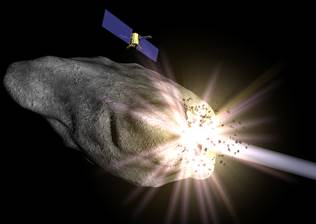
Image courtesy Airbus Space and Defense Germany
The change in the asteroid's momentum caused by such a collision is not all that great, usually some millimeters per second. If applied soon enough, however, it sufficiently alters the orbit of the target asteroid to make it miss the Earth. Although we believe to have the capabilities to avert potentially disastrous asteroid impacts in such a way, only the realization of an asteroid deflection demonstration missions can show that this is actually the case. One of the top priorities of such a deflection demonstration mission is, of course, that a harmless asteroid should not end up close to the Earth some time later. Large uncertainties in an asteroid's physical parameters as well as the additional uncertainties introduced during a deflection attempt do not make it easy to determine with accuracy where the target asteroid will end up several decades after a deflection attempt. Thus, my collaborators and I investigate long term impact risks of potential targets before and after a simulated mitigation demo-missions. Taking the uncertainties most relevant for the deflection process into account we can assess which demonstration missions are safe and which are not. If you are interested in more details, please have a look at my publications on this subject.
Habitability of Planets in Binary Star Systems
In spite of the recent demotion of Pluto, the number of known planets has drastically increased over the last decades. Today, there are thousands of planets confirmed to orbit other stars, and the new generation of ground based and space based telescopes will again increase that number. Since some of the stars out there look like our sun, tempting questions arise: How many of those extra solar planets look like Earth? How many of those are capable of hosting life? Do all potentially habitable, Earth-like planets orbit a sun-like star? Astronomers have already discovered planets that are roughly the size of our Earth orbiting sun-like stars. Yet, we would need to discover a lot more to give accurate statistics on their properties - currently not an easy task. If the sun was an orange, the Earth would only be the size of a pin head orbiting at a distance of 25 meters. Now try to observe the pin head with your backyard telescope when the orange is at a distance comparable to a geostationary satellite... Discovering traces of life on other worlds is even harder. First, we have to understand what to look for. The current consensus is that life as we know it (which is really the only thing we can go by) seems to be comfortable when there is liquid water. Therefore, we search for terrestrial planets that are "habitable" meaning that they are capable of retaining liquid water over a long time. Fortunately, research in climatology, geophysics and astrophysics can be combined to put constraints on where to look for such planets. Areas around a star where the planet would receive too much or not enough sunlight to stay temperate can then be excluded from searches. Studying the star-planet interaction is already challenging for one planet around one star. Why make things even more complicated by adding another sun to the equation? Well, a lot of sun like stars prefer company. Some estimates claim that almost half of the solar analogue stars in our galactic neighborhood have at least one stellar companion. Consequently, I have looked into where habitable planets can be expected to pop up in double star systems. It turns out that one has to study how planets move in such systems in order to give an accurate answer to this question. This leads to so-called "dynamically informed habitable zones". Using this framework, I was able to show that planets in binary star systems can indeed host liquid water. Please see my publications for more details.
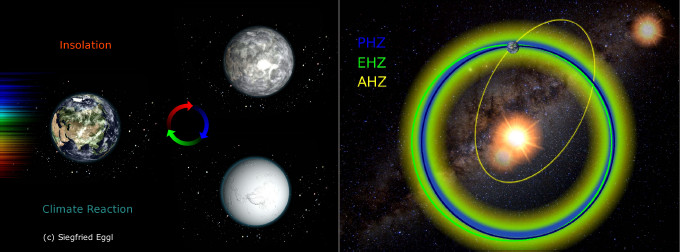
The amount of sunlight an Earth-like planet receives is one of the dominant factors for climate evolution. In double star systems, the planet's orbit cannot stay constant (e.g. circular) for all time due to the gravitational interactions with the two stars. Changes in the planets orbit will influence the amount of insolation (incident sunlight) and hence change the planet's climate. Dynamically informed habitable zones can be used to understand where terrestrial planets can be expected to host liquid water.